Exchange interaction
What is the exchange interaction?
The exchange interaction stabilises the aligned elementary magnets, i.e. the atomic spins, in magnetic materials. This is the only reason why the parallel alignment of the elementary magnets in ferromagnets is so stable and why the magnetic forces between different magnets or between ferromagnetic iron and a magnet are so strong. The physical cause of the exchange interaction is the Pauli exclusion principle.Table of Contents
The exchange interaction manifests itself as a force that acts between elementary magnets,
namely electron spins,
in a solid-state body.
The exchange interaction is a force that can only be understood through quantum theory and is based on the Pauli principle.
It is not directly involved with the magnetic moment
of the electron spins and is not simply a force that arises when an elementary magnet in the material exerts magnetic forces
on a neighbouring elementary magnet.
These magnetic forces would be far too small to explain the strong exchange interaction in ferromagnets.
The forces that follow the Pauli exclusion principle can be enormous.
These forces are even strong enough to stabilise neutron stars against gravitational collapse.
Only the force of a supernova, i.e.
an exploding star that leaves behind a black hole, can overcome the forces of the Pauli principle.
Electrons are so-called fermions.
According to the Pauli exclusion principle, they cannot be in the same place if they do not differ in any other parameter (such as the direction of the spin, for example).
This is what the Pauli exclusion principle states about electrons.
Origin of the exchange interaction
The exchange interaction occurs because the different electron spins in a ferromagnetic material must not differ in the direction of the spin. This is because the Pauli exclusion principle is determined by the symmetry properties of the fermions, i.e. the electrons, which can have different consequences for the orientation of the spin (two electrons in the same space must have opposite spins, whereas two electrons in a ferromagnetic material must not have opposite spins). Just as the Pauli exclusion principle in ferromagnets prohibits the spins of neighbouring electrons from being in opposite directions, a force acts between the electrons to stabilise the parallel position of the spins. This force is called the exchange interaction (see below for details).Consequences of the exchange interaction
In the following, the consequences of the exchange interaction will be discussed first.The electron spin carries a magnetic moment.
This magnetic moment is aligned by an external magnetic field.
If there are unpaired electron spins on the individual atoms in a solid-state body (such as in paramagnets
and ferromagnets), a magnetisation of the entire solid object occurs due to a parallel positioning of the magnetic moments of all atoms and because the contributions of all unpaired electron spins in the parallel position add up to a magnetisation of the entire solid object.
In the case of paramagnets, the exchange interaction between the aligned electron spins is much smaller than the thermal energy, i.e.
the kinetic energy, of the electrons involved.
As a result, the electron spins of a paramagnetic material do not remain permanently aligned at room temperature.
In paramagnets, the magnetisation is lost after the external magnetic field is switched off because the stabilisation of the aligned electron spins as a result of the exchange interaction is not greater than the thermal energy of the electrons.
In ferromagnetic materials, however, the magnetisation remains even after the external magnetic field is turned off.
Consequently, the exchange interaction in ferromagnets is greater than the thermal energy.
A magnetised ferromagnet is not easily demagnetised again.
Only heavy impacts, high temperatures above the Curie temperature
or an oppositely polarised external magnetic field of coercivity can overcome the exchange interaction of the electron spins and thus the magnetisation.
Phenomenon of Weiss domains
Interestingly, a ferromagnet does not magnetise spontaneously, but the electron spins are aligned within certain areas of the material, whereby the electron spins within such an area are aligned parallel to each other. These areas are called Weiss' domains.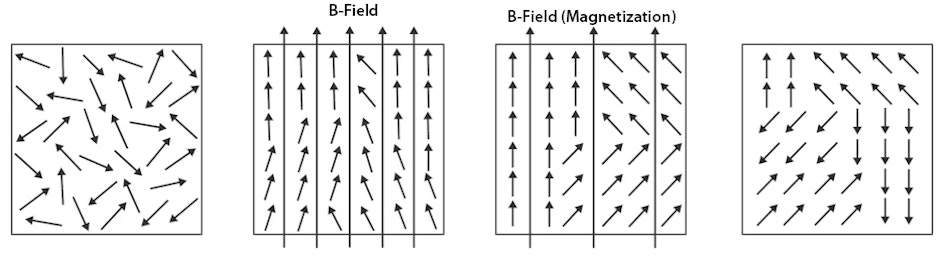
In the image, the magnetic moments of the electron spins are represented by small arrows.
Long arrows that extend beyond the boundary of the images indicate a resulting magnetic flux density in the entire material, i.e.
a magnetic field.
In this case, we speak of a macroscopic flux density.
Without an external magnetic field, the spins of a paramagnetic material are randomly orientated (left image).
The overall magnetisation is zero.
An external magnetic field causes the spins to align, i.e.
magnetisation occurs (second image from left).
In ferromagnets, the spins are aligned completely parallel in large areas, and part of the magnetisation remains even after the field is switched off, thanks to the exchange interaction (second image from the right).
Demagnetisation processes manifest themselves by the fact that the spins start to jumble until the contributions of the individual spins compensate each other.
Nevertheless, the spins remain aligned in parallel in large areas (i.e.
the Weiss domains) (far right).
Often, the alignment of all electron spins in a Weiss domain changes collectively.
The phenomenon of Weiss’ domains, which arise due to the exchange interaction, can be illustrated in a macroscopic model.
For this purpose, a set of compass needles is mounted on a board so they can freely rotate, and then it is observed how they influence each other.
This model also corresponds to the illustration above.
In an experiment, all compass needles can be aligned by an external magnetic field.
Due to the influence of temperature (movement of the compass needles) or external mechanical influence (knocks on the board), entire groups of compass needles can change their orientation.
However, the compass needles within such a group often remain aligned in parallel.
This collective behaviour can be directly observed in electron spins.
Those are actually jumps in the alignment of the electron spins within an entire group of electrons.
In electron spins, this is referred to as Barkhausen jumps.
The area of such a parallel aligned group is a Weiss domain.
The reason for their collective motion is the mutual interaction, the exchange interaction.
In terms of energy, it is more favourable if a whole group of electron spins changes its orientation at the same time than each electron spin doing it individually.
In the experiment, Barkhausen jumps occurring in ferromagnets can be made audible with the help of an amplifier and a loudspeaker.
The Barkhausen jumps manifest themselves as a "crackling sound" in the loudspeaker because the magnetic field on the surface changes slightly during a Barkhausen jump and induces a short current pulse (see chapter on the Barkhausen jump).
Weiss domains can also be observed directly.
In an experiment, fine ferromagnetic dust can be placed on a magnetised material.
This dust then arranges itself mostly along the boundaries between different Weiss domains, forming dark lines in the process (see illustration on the right).
When the Weiss domains shift, a shift of these area boundaries is observed (Barkhausen jump).
At first, it does not seem surprising that neighbouring electron spins interact since the magnetic moments of the electron spins influence each other, and, as such, one could assume that the magnetic field of an electron spin affects the magnetic field of a neighbouring electron spin.
This is what happens in the compass needle model.
However, it can be demonstrated that this magnetic force is far too small to explain the strong stabilisation of electron spins against thermal motion in ferromagnets.
It is not the magnetic forces but the exchange interaction that stabilises the parallel position of the electron spins.
The significance of the Pauli principle for the exchange interaction
As already mentioned, the exchange interaction is based on the Pauli exclusion principle. The Pauli principle is of fundamental importance. It is based on symmetry considerations. More precisely, the Pauli principle must be understood to mean that the wave functions of neighbouring electrons in a solid-state body must be antisymmetrical to each other. This means that the electrons may differ in exactly one or three properties (if all other properties are "symmetrical"), but not in two properties. Because the product of two antisymmetric wave functions is symmetric again.Strictly speaking, an odd number of functions must be antisymmetric if all other functions describing the properties of the particles are symmetric.
The neighbouring electrons in a solid are electrons with an antisymmetric position wave function.
All other functions are symmetrical.
One way to imagine this is that the electrons differ in their position, but not in any other parameter.
Therefore, the wave function that describes the spin must also be symmetrical.
Consequently, the electrons in a ferromagnet must not differ in their spin.
For the same reason that electrons in the same position within an atom cannot have the same spin, the electrons of neighbouring atoms in a solid ferromagnetic object must not have different spins.
This is why the electron spins in a ferromagnet stabilise each other based on the Pauli exclusion principle.
A single electron spin cannot simply flip.
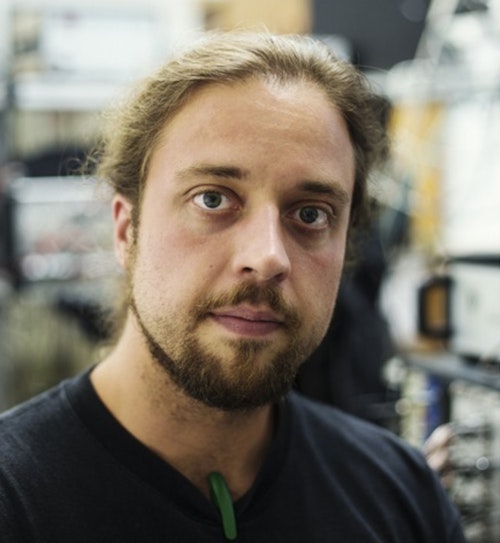
Author:
Dr Franz-Josef Schmitt
Dr Franz-Josef Schmitt is a physicist and academic director of the advanced practicum in physics at Martin Luther University Halle-Wittenberg. He worked at the Technical University from 2011-2019, heading various teaching projects and the chemistry project laboratory. His research focus is time-resolved fluorescence spectroscopy in biologically active macromolecules. He is also the Managing Director of Sensoik Technologies GmbH.
Dr Franz-Josef Schmitt
Dr Franz-Josef Schmitt is a physicist and academic director of the advanced practicum in physics at Martin Luther University Halle-Wittenberg. He worked at the Technical University from 2011-2019, heading various teaching projects and the chemistry project laboratory. His research focus is time-resolved fluorescence spectroscopy in biologically active macromolecules. He is also the Managing Director of Sensoik Technologies GmbH.
The copyright for all content in this compendium (text, photos, illustrations, etc.) remains with the author, Franz-Josef Schmitt. The exclusive rights of use for this work remain with Webcraft GmbH, Switzerland (as the operator of supermagnete.hu). Without the explicit permission of Webcraft GmbH, the contents of this compendium may neither be copied nor used for any other purpose. Suggestions to improve or praise for the quality of the work should be sent via e-mail to
[email protected]
© 2008-2024 Webcraft GmbH
© 2008-2024 Webcraft GmbH